Plate tectonics is the fundamental mechanism that drives geological processes in the geosphere. Plate tectonic theory is based on an understanding of the Earth's internal structure, the different types of tectonic plates and plate boundaries, and the driving forces of plate movements. The occurrence of earthquakes and volcanoes, the distribution of different rock types, and the Rock Cycle, as well as the processes of mountain building, continental rifting and seafloor spreading, can be concisely explained by plate tectonic processes.
Structure of the Earth
The Earth is an irregular sphere, with a radius that varies between 6,356 and 6,378 kilometres. This solid sphere is chemically divided into layers that become less dense from the centre towards the surface. Three main layers are recognised: the core (which comprises an Inner Core and an Outer Core), the mantle, and the crust. Each layer has a distinctive chemical composition, and a different density (Figure 1).
Figure 1: Generalised structure of the Earth.
Scientists infer that the dense core is primarily composed of the heavy elements iron and nickel. The outer core is made of molten iron, which produces the Earth's magnetic field.
Surrounding the core is the less-dense mantle, which extends to a depth of about 2,900 kilometres. The mantle is rich in iron- and magnesium-bearing silicate minerals.
The outer layer of the Earth is termed the crust, which is divided into oceanic crust and continental crust. Overall, continental crust is richer in the element silica, and is less dense, than oceanic crust.
- Oceanic crust (about 10 km thick) is composed of iron-, magnesium-, calcium-, and aluminium-rich silicate minerals that typically form a dark coloured, heavy rock called basalt.
- Continental crust (about 20 - 60 km thick) is composed of potassium-, sodium-, and aluminium-rich silicate minerals that form a diverse range of rock types such as granite.
Dynamic Structure of the Earth
The crust and upper part of the mantle of the Earth is further subdivided into the lithosphere and the asthenosphere (Figure 2). The slow movement of the lithospheric plates over the mobile asthenosphere is known as plate tectonics, a process that maintains the surface of the Earth in a dynamic and active state.
Figure 2: Generalised structure of the crust and upper mantle.
- The lithosphere is a strong layer, extending to a depth of 100 to 150 kilometres, that comprises the crust and part of the upper mantle. Over the surface of the Earth, the lithosphere is separated into seven large plates, and several smaller plates. These plates, which terminate at different types of plate boundary, move over the underlying asthenosphere.
- The asthenosphere is a weaker layer, extending from approximately 150 to 400 km depth, upon which the lithospheric plates move, and from which magmas that form the oceanic crust are derived. Heat from the Earth's core creates circulation patterns (convection currents) in the mantle that drive the motions of the overlying plates.
What drives Plate Tectonics?
Plate tectonics is driven by the internal heat energy of the Earth. This comprises the heat left over from the initial formation of the Earth, combined with heat from the decay of radioactive minerals contained in the rocks.
Heat from the Earth's lower mantle rises as plumes towards the upper mantle where cooling occurs. The plumes spread out, then sink back into the interior (Figure 3). This process is known as mantle convection. These convection currents propel the motion of plates.
Although heat drives mantle convection and the motion of plates, the mantle is mostly solid. The rock forming the mantle, however, behaves in a semi-plastic manner, which enables the slow transfer of materials.
Figure 3: Mantle convection as the driving force for plate tectonics.
Types of Plate Boundary
There are three types of plate boundary: convergent, divergent, and transform plate boundaries.
Divergent plate boundaries
(Figure 4a): occur where two lithospheric plates move away from each other, driven by magma rising from deep within the mantle. Volcanic activity at a divergent plate boundary creates new lithosphere along what is known as a spreading ridge.
Convergent plate boundaries
(Figure 4b): occur where two lithospheric plates move towards each other, with one plate overriding the other. The overridden plate is driven back into the mantle, and is subsequently destroyed along what is known as a subduction zone. During this process, earthquakes and volcanic activity are generated in the overriding plate.
Transform plate boundaries
(Figure 4c): occur where two lithospheric plates slide laterally past each other. Earthquakes are generated along this type of plate boundary. Importantly, lithosphere is preserved along transform boundaries, it is not created or destroyed as it is at divergent and convergent plate boundaries.
Plate Tectonics and Earth Surface Processes
Plate tectonics is a process that maintains the surface of the Earth in a dynamic and active state. Consequently, it drives such processes as:
- Magmatism: the primary way in which new elements and new materials are transported as molten rock (magma) from the mantle to the crust. This is the first stage in the process known as "The Rock Cycle". The molten rock (magma) issuing from cracks in the surface of the Earth cools to create new rocks, and thereby introduces new elements and minerals into the rock cycle. These new rocks are broken down (weathered), transported (eroded), accumulated (deposited), and buried (lithified) to form other rocks, which are subsequently uplifted and then weathered, eroded and deposited in a repeated cycle.
- Plutonism: a large proportion of the mobile magma never reaches the Earth's surface. This magma accumulates and cools in the crust to form bodies of new rock called plutons.
- Volcanism: at certain types of plate boundary, magma and gases escape at the Earth's surface, either explosively, as ash, or effusively, as lava forming volcanoes.
- Earthquakes: the sudden displacement of rocks along faults, triggered by plate movements, or by the movement of magma upwards in the crust, causes violent shaking of the Earth (Figure 5).
- Folding and Faulting: movement of the lithospheric plates bends and fractures the crust, creating folds and faults in the rocks.
- Mountain Building: along certain convergent plate margins, prolonged movement of the lithospheric plates causes crumpling of rocks between the plates, which leads to the creation of mountain chains and volcanoes (Figure 6).
- Opening of Ocean Basins and Rifting: Behind the chain of volcanoes adjacent to convergent plate margins, tensional tectonic forces develop due to slab pull. This can lead to development of a back-arc rift zone. At these sites, a new oceanic spreading centre may develop, leading to formation of new oceanic crust (Figure 7).
Within regions of continuous continental crust, the process of mantle upwelling leads to thinning of the crust and to the development of rift valleys. The Great African Rift Valley in Kenya is an example of this type of continent-continent breakup.
Magmatism
Magma is derived from deep within the Earth by partial melting of the upper mantle or lower crust. It is composed of a high temperature mixture of silicate material (magmatic liquid), water, and dissolved gases. Magma may also contain crystals that formed during cooling of the magma, and rock fragments incorporated into the magma during its ascent towards the Earth's surface.
Magmatism is the process by which molten rock (magma) rises to the upper crust where it may:
- Either, cool and crystallize relatively slowly to form intrusive igneous rock (plutonic rock);
- Or, be erupted from volcanoes at the Earth's surface in the form of lava and ash that cool relatively quickly to form extrusive igneous rock (volcanic rock).
Solid mantle material rises close to the Earth's surface at divergent plate boundaries (Figure 8). This process is accompanied by a decrease of pressure, which lowers the melting temperature of the mantle material. Thus, when the solid mantle reaches a certain level, it begins to partially melt to form a magmatic liquid. Buoyancy causes the magmatic liquid to rise towards the Earth's surface, away from the zone of melting, leaving behind unmelted solid material. Partial melting, caused by the reduction in pressure associated with the upward movement of mantle, is termed decompression melting.
Figure 8: Plate tectonic settings.
Decompression melting is also responsible for the production of magma at hotspots (Figure 8). A hotspot is a location where an active convection system occurs within the mantle. At these locations, the solid mantle materials are hotter and less dense, and are moving upwards towards the crust in plumes.
At convergent plate boundaries, the subducting plate is heated as it descends, releasing hydrous fluids into the plate above (Figure 8). The injection of these fluids into the overlying lithospheric mantle causes a lowering of the melting point of the mantle materials, which partially melt to form magma. These magmas rise to the surface through the crust, feeding the volcanoes that occur in both island arc and continental margin arc settings. For example, the chain of volcanoes along the convergent plate boundaries surrounding the Pacific Ocean basin is known as the "Ring of Fire" (Figure 9).
Figure 9: Active volcanoes, plate tectonics and the "Ring of Fire".
Volcanoes in different Plate Tectonics Settings
Magma erupted from a volcano is generally a mixture of three main components, crystals, magmatic liquid, and dissolved gases (mostly water vapour). Different types of volcanoes occurring in contrasting plate tectonic settings will exhibit varying degrees of explosivity depending upon the composition of the erupting magma, in particular the dissolved gas content. When a magma is erupted at the surface, any dissolved gas comes out of solution and is released in the form of gas bubbles.
Around the world, a complete spectrum of magma compositions can be found. These range from magmas rich in magnesium and iron aluminium silicate minerals (basalt), to those rich in sodium, potassium, and calcium aluminium silicate minerals (rhyolite), with a range of intermediate magmas occurring in between (e.g. andesite).
The magma in basaltic volcanoes is relatively hot, and flows easily. This allows any gas bubbles to escape, resulting in largely non-explosive eruptions. In contrast, the magma in rhyolitic volcanoes is relatively cooler and more viscous. Any gas bubbles in the rhyolitic magma have great difficulty escaping, and are explosively released, shattering the magma. Thus, rhyolitic volcanoes are more explosive than basaltic volcanoes, producing greater quantities of ash. Volcanoes that generate roughly equal proportions of lava and ash produce the characteristic stratovolcanoes that occur around the Pacific "Ring of Fire" (Figure 10).
Three common types of volcanoes that occur in different tectonic settings are shield volcanoes, stratovolcanoes, and caldera volcanoes.
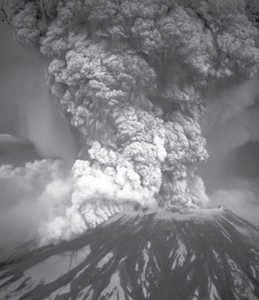
Figure 10: Mount St. Helens volcano, USA
(Photo courtesy of USGS/
Cascades Volcano Observatory).
Shield Volcanoes
Most basaltic eruptions in intraplate or mid-ocean spreading centre settings form low surface slope shield volcanoes. Typically, lava fountains from the vent in these eruptions (Figure 11). Large volumes of low viscosity basaltic magma pour out as rivers of lava. This style of eruption is characteristic of Hawaiian-type volcanoes.
Stratovolcanoes
Volcanoes associated with continental margin arc or island arc tectonic settings are dominantly andesitic or rhyolitic in composition. These volcanoes typically form stratovolcanoes (composite volcanoes), and the eruption style is generally more explosive than Hawaiian-type volcanoes. A typical stratovolcano has a steep cone shape, made up of alternating layers of ash and lava (Figure 12).
Depending upon the amount of dissolved gas contained in the erupting magma, the eruptions at stratovolcanoes may vary from minimally explosive (Strombolian-type) to highly explosive (Plinian-type). The greater the dissolved gas content, the more explosive an eruption will be, and the larger the volume of erupted volcanic ash.
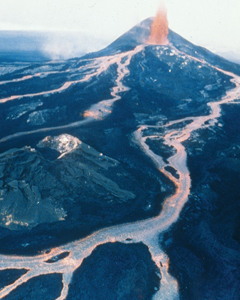
Figure 11: Kilauea volcano, Hawaii
(Photo countesy of the USGS/
Cascades Volcano observatory).
Figure 12. Generalised structure of a stratovolcano and associated volcanic hazards.
Caldera Volcanoes
Rhyolitic volcanoes in convergent boundary tectonic settings may form caldera volcanoes. Caldera volcanoes are ones in which the top part of the volcano has been explosively destroyed following collapse into an underlying drained magma chamber (Figure 13). Caldera volcanoes are the result of very violent eruptions.
The volcanic rocks that occur in Hong Kong are predominantly of rhyolitic composition, consisting mainly of ash. These rocks were formed by violent eruptions of ash from caldera-type volcanoes. Ash clouds from caldera-type volcanoes are commonly so large, that they collapse under gravity and flow over the landscape like a fiery fluid.
Figure 13. Formation of a caldera volcano -
(A) Explosive eruption partially empties the underlying magma chamber;
(B) The summit of the volcano collapses into the empty space left in the magma chamber, forming a caldera.
Volcanic Hazards
Hazards created by volcanoes can be divided into those that are produced directly from volcanic activity (primary hazards), and those that are produced indirectly from volcanic activity (secondary hazards) (Figure 12).
Primary hazards include: lava flows, pyroclastic flows, ash-falls, and gas emissions.
Secondary hazards include: lahars (mud flows), floods, fires, and tsunami (large sea waves).
Earthquakes
Earthquakes are associated with all types of plate boundary. They result from the sudden release of energy that occurs when the stress that builds up between two adjacent, moving tectonic plates finally overcomes the friction that holds the two plates together (Figure 14).
Figure 14. Seismic waves generated by movement along a fault deep underground.
At transform plate boundaries, such as the San Andreas Fault in California, lateral displacement between two adjacent plates may occur in sudden catastrophic movements that leads to splitting of the Earth's crust at the surface. These ruptures in rock are known as faults.
At divergent or convergent plate boundaries, the movement between the tectonic plates may occur at any depth below the Earth's surface. However, some earthquakes that occur in subduction zones may be associated with faults that reach the surface. In some cases, these can result in catastrophic vertical movements of the ground and the generation of tsunamis, such as the one that occurred following the December 2004 Indian Ocean earthquake.